Contrary to the common misconception that membranes exist as simple, flat lipid bilayers, membranes are actually often found as highly curved structures composed of various lipids and proteins. The distribution and function of lipids and proteins give membranes their shapes but also enable cells to undertake a wide array of functions. Cell membranes can look like tubes, vesicles, or cristae but can also form dynamic curved structures that enable cell migration, protrusion formation, and viral infection, among other functions.
Many biophysical studies have delved into the molecular details of membrane curvature and the specific distribution and role of proteins and lipid species. Recognizing the challenge in navigating through the multitude of existing studies on this subject, the Research highlight
“Membranes get in shape: Biophysics of curving bilayers” by Shurer and Levental covers Biophysical Journal studies published on this topic in the years 2021 and 2022. Shurer and Levental present a series of studies on the biophysics of membrane curvature, divided into four main sections that offer insights into the molecular characteristics of curved membranes.
Lipid sorting in curved membranes
Shurer and Levental first explore how lipid molecular shapes arise and the resulting link between membrane curvature and lipid sorting. As illustrated in Figure 1, lipid shape results from the ratio of the lipid headgroup volume relative to the volume occupied by their fatty acid chains. Cylindrical lipids like phosphatidylcholine and phosphatidylserin, with similar headgroup and acyl chain volumes, prefer flat membranes. Because of their small heads and larger tails, conical lipids like phosphatidylethanolamine prefer negatively curved membranes. Conversely, lipids that have larger heads and smaller tails, like lysophosphatidylcholine, have an inverted conical shape and in turn favor positive curvatures.
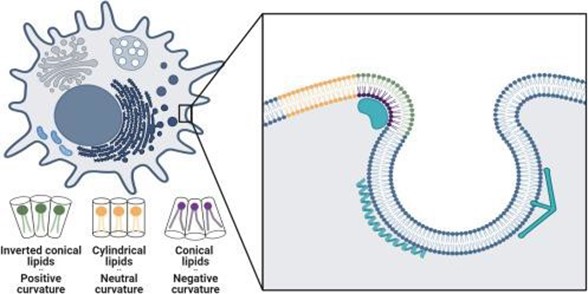
Figure 1: Schematic illustrating a cell (top left) with its different curved membranes and close-up view (inset) of lipid and protein sorting within the cell’s curved membrane. Individual lipid shapes and their corresponding curvatures are denoted in green, yellow, and purple (with detailed representations at the bottom left). Adapted from Shurer and Levental, 2024.
However, individual lipid curvature is not the only factor that determines membrane shape. In the study by Cino and Tieleman, the authors use coarse-grained dynamic simulations to explore the effect of curvature on lipid sorting in buckled asymmetric bilayers. This study reveals differences in lipid partitioning between the outer and inner leaflets of the bilayer, which suggests that membrane asymmetry and curvature may also drive lipid clustering. Another aspect that Shurer and Levental discuss is how existing experimental models often over-simplify lipid interactions, such that interacting lipids are assumed to contribute only linearly to membrane properties. In contrast, the study by Lessen et al., by using all-atom molecular dynamics simulations, found that certain lipid dimers also exhibit nonadditive curvature preference.
Packing defects in curved membranes
Next, Shurer and Levental explore the link between membrane curvature and hydrophobic packing defects, specifically addressing how curvature affects the number and size of these defects. Proteins that have amphipathic helices (AHs) can insert into membranes via hydrophobic defects that either stabilize or destabilize membrane curvature. Several studies using diverse methodologies have explored these interactions. The protein Sar1 is provided as one example by Auerswald et al., who combine Langmuir film balance and confocal fluorescence correlation spectroscopy to quantify its insertion into a membrane leaflet. The study by Surmeier et al., on the other hand, used x-ray reflectometry measurements to determine the insertional depth of various viral (class I–III) fusion proteins, elucidating the influence of peptide insertional depth on curvature formation.
Other studies, by Courtney et al. and Liang et al., also cover the role of synaptic vesicle fusion to the plasma membrane, regulated by SNARE proteins that contain AH domains. These studies emphasize the role of membrane shape in regulating neuronal communication. Computational simulations have also been used to elucidate these interactions. Notably, the study by Mandal et al. compares three versions of the coarse-grained MARTINI model, emphasizing that protein insertional depth does, in fact, influence curvature preferences for certain AHs.
Protein sorting in curved membranes
Beyond the association of AHs at curved membranes, as discussed above, curved membranes also sort proteins through other mechanisms. Protein diffusion, for instance, is a fundamental process for distributing proteins throughout cell membranes. Shurer and Levental highlight the study by Rojas Molinas et al. who used numerical simulations to explore how curved membranes affect protein diffusion. Modeling various membrane-bending geometries, this study observed a slowing down of the diffusion dynamics on strongly curved shapes. This, in turn, results in heterogeneous distribution of proteins on curved membranes.
A known example of proteins sensitive to membrane curvature is the Bin/amphiphysin/Rvs (BAR)-domain-containing family. On the basis of evidence suggesting that inverse BAR protein domains sense and reshape curved membranes, Jarin et al. used highly coarse-grained molecular dynamics simulations to explore this interaction. This study sheds light on how membrane curvature can sort lipid species, which in turn recruit specific proteins into functional ultra-structures. Furthermore, clathrin, a key player in coated vesicle formation and cargo uptake during endocytosis, also exhibits sensitivity to membrane curvature. Uncertain of whether clathrin senses curvature directly or only passively, Zeno et al. explore this mechanism. This study finds that clathrin senses highly curved membranes, a process that is enhanced synergistically by other endocytic curvature-sensing proteins. Thus, interacting proteins may play a cooperative role in sensing the curvature of cell membranes.
Curved membranes must overcome tension
Finally, Shurer and Levental discuss how curved membranes are also faced with the challenge of overcoming membrane tension. This is particularly true for membranes that are densely packed with large, flexible biopolymers tethered to the membrane. By using a generic physics-based membrane polymer model, Kutti Kandy et al. explored the biophysics and mechanobiology of membrane shape regulation by anchored biopolymers. This study found that membranes with lower tension had higher average curvature, suggesting that excess membrane area facilitates membrane bending by biopolymers. Similarly, Liese and Carlson developed a theoretical model to investigate the impact of membrane tension on three different cases of crowding-induced curvature: low, intermediate, and high crowding. This model revealed how various membrane shapes can result from the interplay between protein crowding-induced curvature and membrane tension.
Conclusions
Altogether, the research highlight by Shurer and Levental explores a diverse set of Biophysical Journal studies that shed light on the intricate molecular mechanisms by which curvature governs lipid and protein sorting, offering valuable insights into the resulting properties and morphologies of curved membranes.
As we delve deeper into understanding these processes with biophysical studies, we gain a deeper appreciation for how membrane curvature fundamentally shapes membrane properties, morphologies, and functions. Ultimately, these and future studies in the field can help us uncover the fascinating complexity of curved cellular membranes and their vital roles in cellular physiology and function.